Understanding the molecular structure of SARS-CoV-2
- Felipe Perez
- Jun 2, 2021
- 9 min read
Finding ways to weaken viral spread effectiveness.
by Felipe Perez
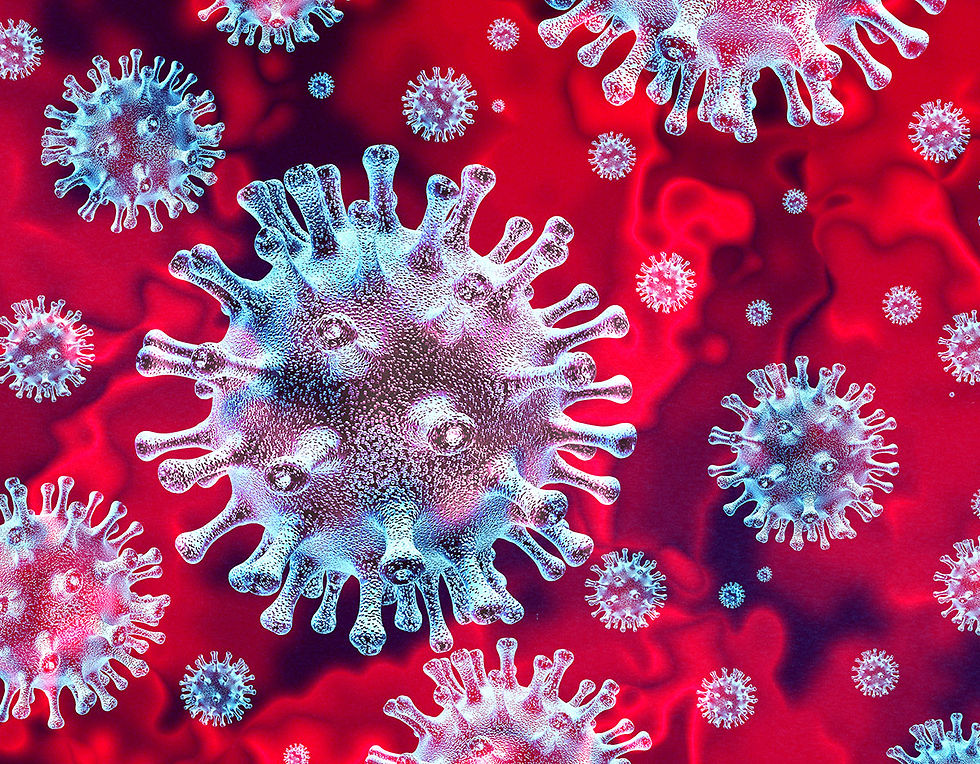
Studies indicate that HCoV-19 is more effective at infecting people than previously known coronaviruses such as HCov-1, probably due to its “spikes” or S protein affinity for one of the most abundant cellular receptors in human cells, the ACE2.
Abstract
The SARS-CoV-2 (also referred to as HCoV-19) is one of the strains of the viruses classified as coronaviruses, SARS stands for severe acute respiratory syndrome and CoV-2 means Corona Virus 2. According to the World Health Organization (WHO), since the start of the outbreak in January of 2020, this virus has infected nearly sixty million people around the world and has killed more than 1.5 million patients by the time this data was collected (December 2020) being the Americas and Europe the most affected continents. The world's scientists are working around the clock trying to decipher the virus, and at the time of this writing there is no available vaccine nor effective medications yet to stop the spreading; but three laboratories have announced successful trials with their vaccines, and the beginning of early administration is expected to commence by the end of December 2020. Several aspects of the molecular components of the virus are still unclear. Scientists are reporting new mutations of the virus as they struggle to stop its spread and governments are trying their best to produce timely policies while supporting their citizens as the quarantine and social distancing measures are in place. Studies indicate that HCoV-19 is more effective at infecting people than previously known coronaviruses such as HCov-1, probably due to its “spikes” or S protein affinity for one of the most abundant cellular receptors in human cells, the ACE2. This paper attempts to explain, in simple terms, the basic molecular structures and principles that SARS-CoV-2 utilizes to attack the human body, aiming to provide information to the community which will help to a better understanding of the importance of utilizing face masks, practicing social distancing and practicing careful hygiene amid the pandemic.
Coronaviruses are given this name because they have a protein coat that projects spike-like structures that resemble a crown, from the Latin term “corona.”
Introduction
Viruses are not classified as living organisms as they lack most of the structures found in cells, as well as the mechanisms for metabolization and replication, namely organelles, ribosomes, and a plasma membrane. Viruses entirely depend on a host cell to replicate and produce more viral particles; they infect and hijack the machinery of bacteria and cells of plants, animals, and fungi. Virions are the smallest units of a complete, infectious virus, which has two main components, the genetic material found in their core, which is either Ribonucleic acid (RNA) or Deoxyribonucleic acid (DNA), and a capsid, an outer protein coat that gives the virus its specificity (Rye et al., 2020). Sometimes virions are surrounded by an outer phospholipid and protein envelope; this membrane is part of their host’s membrane which the virus has modified after taking over the cell. The shape adopted by a virus is defined by the arrangement of the subunits of its capsid, called capsomeres, and even though there are several ways to classify viruses, the shape they adopt, is one of the characteristics commonly used by scientists to classify viruses into four major categories: filamentous, isometric, enveloped, and head and tail. Filamentous viruses (Figure 1A) such as the tobacco mosaic virus, TMV, exhibit a rod-like structure and are commonly found infecting plants. Isometric viruses (Figure 1B) are normally spherical shaped; herpes and human rhinovirus are examples of this last group. The head and tail group (Figure 1C), holds viruses with more complex shapes, combining spherical heads attached to filamentous-like tails that attack mostly bacteria, they are also known as bacteriophages. Enveloped viruses (Figure 1D) are the ones with a surrounding membrane over the capsid, these viruses are most commonly seen infecting animal cells, and it is in this group where we can find HIV and the current pandemic-causing Novel Coronavirus-19 (Rye et al., 2020).
Coronaviruses are given this name because they have a protein coat that projects spike-like structures that resemble a crown, from the Latin term “corona” (Fig. 2) (Singhal et al., 2020). The virus utilizes these spikes to bind with the receptors of the cell which are transmembrane proteins with a domain facing the extracellular space. The virus then enters the cell to release its genetic material and take over the cell's machinery. The following section of this article focuses on studying and understanding the molecular structure of this new type of coronavirus. Making it understandable for the general public, and working as a universal collaborative network with science and the public, the knowledge obtained is going to help the scientific community around the world to identify, design, and implement better ways of diagnosing, and treating the disease associated with the virus to help the purpose of prolonging life and wellness of all human beings.
Background and discussion
Recent studies suggest that the distinctive spike-shaped (S) protein of the virus is what renders the novel SARS-CoV2 more infectious and more pathogenic than its closest relatives (South et al., 2020). These spikes of the SARS-CoV-2 are optimized to interact with a cell receptor called Angiotensin Converting Enzyme 2 (ACE2) which is found in many tissues of the body including the epithelium lining the respiratory system. The SARS-CoV-2 has an incubation period of 1-15 days, but infected patients remain asymptomatic for the first 3-5 days on average, increasing the transmissibility rate (Yang & Wang, 2020). The SARS-Cov-2 genetic material is composed of a single strand of positive-sense RNA with a 5' cap and a 3' polyA tail. This means ribosomes can attach to the RNA immediately and its genome can be translated directly from the single strand into proteins, without needing any further processing (Chen et al., 2020). This is important because eukaryotes need to modify mRNA's 5' and 3' ends to create the cap and tail respectively before translation can begin. The SARS-CoV-2 genomic code is 29,811 nucleotides long, and it is the instruction booklet that directs the production of proteins necessary for the virus to replicate. Figure 3 (Astuti and Ysrafil 2020) portraits the segments that encode for each of the proteins. Some of these proteins are structural proteins (sps), and the main four are S, M, N, and E, corresponding to Spike, Membranes, Nucleocapsid, and Envelope, respectively. The S protein is a glycoprotein that, as mentioned previously, mediates the virus’ entry to the host cell by binding to the ACE2 receptor. The receptor-binding domain (RBD) found in the SARS-CoV-2 S protein, has mutated from the previous coronaviruses and is composed of six amino acids that have the greatest affinity for the ACE2 receptors of humans, cats, and other mammals, compared to those of the other beta +ssRNA coronaviruses seen before (Andersen et al.,2020).
The M protein is described as a three transmembrane domain structure that binds and stabilizes the membrane to the nucleocapsid and shapes the virion to the characteristic spherical form it resembles. The N protein (nucleocapsid) is physically bound to the viral RNA in the endoplasmic reticulum-Golgi region. The main function of this protein is to protect the viral genome by recognizing and associating with the newly transcribed RNA strand and coiling up to form oligomers that make up the capsid, which will be enclosed in the core of the virus where it will be protected from the host’s immune system. The E protein, which is about 76-100 amino acids long, is the smallest viral protein, and its main functions are related to virus assembly, maturation, and release (Chen et al., 2020). The E protein is integral to the membrane of the virus showing two water affinities, one hydrophilic and one hydrophobic; the hydrophilic ends are smaller and protrude in and out of the membrane, whereas the hydrophobic body is the largest portion made up of amino acid residues which constitute the transmembrane domain. The viral genome also serves as a template for replication and translation for other accessory proteins called 3a, 6, 7a, 7b, 8, and 10. Although these proteins have not been fully characterized, scientists think they are not essential for the viral replication cycle, but rather, are involved in the virus-host interactions and can give important clues about SARS-CoV-2 virulence (Schoeman & Fielding, 2019).
The viral cycle begins after the spikes (S protein) attach to the ACE2 receptors of the host cell, and the type II transmembrane serine protease (TMPRSS2) from the cell membrane activates the receptor site of the S protein. This activation enables temporary structural modifications in both the cell membrane and the ends of the “spikes”, granting the virus access to enter the cell by a phagocytic process. Research shows that goblet and ciliated cells of the nose express higher amounts of surface ACE2, making this an accessible port of entry for the virus into the human body (Astuti and Ysrafil, 2020). Once the virus is inside the cell, the ribosomes of the host translate the viral RNA strand to produce the RNA polymerase specific for the viral genome (RNA) to be replicated; the ribosome continues translating the viral RNA to produce proteins S, E, and M proteins for later activation. Once the proteins have undergone a series of modifications, they get packed into vesicles and leave the endoplasmic reticulum (ER) towards the Golgi apparatus (GA) to be stored and maturated for later release through exocytosis so the newly formed virions can go and infect other cells and other hosts (Fehr & Perlman 2015).
As we understand the origins of these types of viruses, we can relate their mutations and spread to the tight human interactions with animals.
Conclusion
The SARS-CoV-2 has evolved into a pandemic that is re-shaping the way we live, work, and communicate. It is of vital importance to understand the molecular structure of SARS-CoV-2 for a better understanding of the infectious processes and how the virus interacts with the cell. By knowing the highly specific structures of the virus, scientists can develop antibodies that bind to the viral spikes, inactivating and making the virus innocuous to the human population, or synthesizing new medications to destabilize the nucleocapsid causing the virus to disassemble; or medications can be developed that make the RNA unachievable, or that inactivate the E protein to keep the new virions from being released, etc. As we understand the origins of these types of viruses, we can relate their mutations and spread to the tight human interactions with animals. As we live in an industrialized world, we depend more and more on animals for food, work, and research. Among others, climate change has played an important role in merging human communities and animals to share habitats. These interactions have brought us even closer to animals forgetting that sometimes, illnesses can be caused by cross-species transmission or “spillovers”; such is the case of the zoonotic viruses such as HIV, MERS, rabies, and bacteria causing leprosy, and, today, Covid-19, which is the third major outbreak caused by coronaviruses in the last decade. This spillover has taken the lives of thousands of people, much more than the MERS-CoV and SARS-CoV-1 did, meaning these viruses are more infectious each time. Scientists are sure the coronaviruses are evolving and adapting to infect human cells which is why we need to understand the molecular structure of the virus and restructure the way we interact with animals and prepare for pandemics. Furthermore, by making the general public aware and providing appropriate educational material that explains the structure and mechanisms used by the virus to infect humans, communities can step up to help scientists and health care workers to stop the pandemic by simply using face masks correctly, washing hands often, and practicing social distancing.
References
Andersen, K., Rambaut, A., Lipkin, W. I., Holmes E., & Garry R. F. (2020). The proximal origin of SARS-CoV-2. Nat Med 26, 450–452. https://doi.org/10.1038/s41591-020-0820-9
Astuti, I. & Ysrafil, N. (2020). Severe Acute Respiratory Syndrome Coronavirus 2 (SARS-CoV-2): An overview of viral structure and host response. Diabetes & metabolic syndrome, 14(4), 407–412. Advance online publication. https://doi.org/10.1016/j.dsx.2020.04.020
Chen, Q, Guo, D., (2020) Emerging coronaviruses: Genome structure, replication, and pathogenesis, Journal of Medical Virology Volume: 92, Issue: 4, pp 418-423. https://onlinelibrary.wiley.com/doi/pdf/10.1002/jmv.25681
Dewald, S., & Fielding, B. (2019). Coronavirus envelope protein: current knowledge. Schoeman and Fielding Virology Journal https://doi.org/10.1186/s12985-019-1182-0
Dongwan, K., Lee, Y. J., Yang, Y. S., Kim, J. W., Kim, N., and Chang, H. The Architecture of SARS-CoV-2 Transcriptome https://www.cell.com/cell/pdf/S0092-8674(20)30406-2.pdf
Fehr, A. R., & Perlman, S. (2015). Coronaviruses: an overview of their replication and pathogenesis. Methods in molecular biology (Clifton, N.J.), 1282, 1–23. https://doi.org/10.1007/978-1-4939-2438-7_1
Gorbalenya, E., Baker, S., Baric, S., De Groot R., Drosten, C., Gulyaeva, A., Haagmans, L., Lauber, C., Leontovich A., Neuman B., Penzar, D., Perlman S., Poon, L., Samborskiy, D., Sidorov, I., Sola, I., & Ziebuhr J. (2020). The species Severe acute respiratory syndrome-related coronavirus: classifying 2019-nCoV and naming it SARS-CoV-2. Nature microbiology, 5(4), 536–544. https://doi.org/10.1038/s41564-020-0695-z
Liu, W., Li, H., (2020) COVID-19 Disease: ORF8 and Surface Glycoprotein Inhibit Heme Metabolism by Binding to Porphyrin. ChemRxiv. https://s3-eu-west-1.amazonaws.com/itempdf74155353254prod/11938173/COVID-19_Disease__ORF8_and_Surface_Glycoprotein
_Inhibit_Heme_Metabolism_by_Binding_to_Porphyrin_v2.pdf
Rye, C., Wise, R., Oshkosh, V., Desaix, J., Choi, J., & Avissar Y. (Ed.).(2020). Biology 2e. Openstax https://openstax.org/details/books/biology
Sah, R., Rodriguez A. J., Jha, R., Chu, D. K. W., Gu, H., Peiris, M., Bastola, A., Lal, B. K.,
Ojha, H. C., Rabaan, A. A., Zambrano, L. I., Costello, A., Morita, K., Pandey, B. D., Poon, L. M., (2020) Complete Genome Sequence of a 2019 Novel Coronavirus (SARS-CoV-2) Strain Isolated in Nepal. Microbiology Resource Announcements, 9 (11) https://mra.asm.org/content/9/11/e00169-20
Singhal, T. A Review of Coronavirus Disease-2019 (COVID-19). (2020) Indian J Pediatr 87, 281–286. https://doi.org/10.1007/s12098-020-03263-6
Sohrabi, C., Alsafi, Z., O'Neill, N., Khan, M., Kerwan, A., Al-Jabir, A., Iosifidis, C., & Agha R. (2020). World Health Organization declares global emergency: A review of the 2019 novel coronavirus (COVID-19). International Journal of Surgery https://www.sciencedirect.com/science/article/pii/S1743919120301977
South, A.M., Tomlinson, L., Edmonston, D., et al. (2020). Controversies of renin-angiotensin system inhibition during the COVID-19 pandemic. Nat Rev Nephrol 16, 305–307. https://doi.org/10.1038/s41581-020-0279-4
Yang, P., Wang, X. (2020). COVID-19: a new challenge for human beings. Cell Mol Immunol 17, 555–557. https://doi.org/10.1038/s41423-020-0407-x
Comments