The origins of SARS-CoV-2
- Claire Sansaricq
- Jun 2, 2021
- 11 min read
Updated: Jun 2, 2021
Encroachment on natural wildlife habitats, cross-species viral transfer, and viral mutation.
by Claire Sansaricq
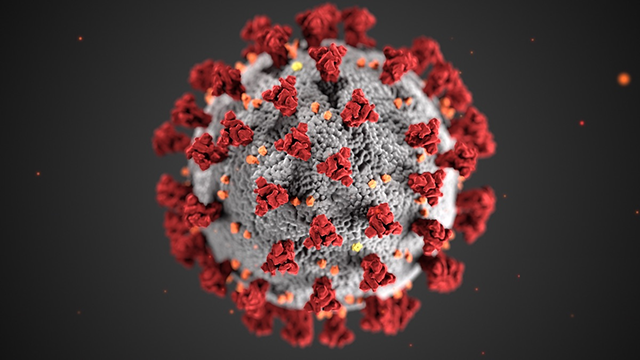
It is vital that we understand that the emergence of SARS-CoV-2; it is not the result of one factor, is the result of many.
Abstract
The emergence of SARS-CoV-2, the virus that causes the infectious disease COVID-19, is the culmination of inadequate systemic developments in infrastructure expansion and its effects on natural wildlife habitats, agriculture, strategic scientific viral outbreak prevention, and global pandemic preparedness. It is vital that we understand that the emergence of SARS-CoV-2; it is not the result of one factor, is the result of many. This paper discusses the relative impact of encroachment on wildlife habitats, expanding bat migration territories, primary viral host carriers and secondary viral host receptor species, cross species viral transmission, and human susceptibility to coronaviruses. Also discussed is the need for further development of comprehensive strategies that will broaden the research and forecasting of viral family mutations. The potential risk of these converging inadequacies must be evaluated and improved upon in order to diminish the impact of future threats.
Many scientists believe that the origins of SARS-CoV-2 trace back to a bat species, known as the Intermediate Horseshoe Bat, Rhinolophus affinis.
Introduction
In late 2019, the world was taken by surprise when the city of Wuhan, China, became the launching point for what would become one of the most devastating viral pandemics of all time. Since its emergence, SARS-CoV-2 has exposed every weakness of the global health care community, and the insufficient systemic capability of every country in the world to face this crisis. This highly contagious, deadly, and sometimes asymptomatic viral infection had already spread to thousands of people in China before the devastating nature of COVID-19 was reported to the World Health Organization. This massive head start paired with poorly applied strategic emergency response and pandemic prevention tactics created a domino effect that would quickly incapacitate healthcare systems and effectively lead to a global economic shutdown. In the past, the world had seen the deadly potential of other coronaviruses such as SARS-CoV-1 (Severe Acute Respiratory Syndrome Coronavirus 1), MERS (Middle East Respiratory Syndrome), and the ever-evolving cold and flu. However, the unique nature of SARS-CoV-2 has baffled healthcare experts. One of many problems that makes this novel virus unique is the wide range of symptoms suffered by people who have become infected (Shereen, M., et al, 2020). In some patients, particularly those over the age of 40 with pre-existing health conditions, including diabetes, lung disease, and cardiac issues, the virus has had a catalytic effect on the human immune response. The most critical cases lead to inflammation of the bronchi along with a dangerously high accumulation of mucus in and around the alveoli, inhibiting the exchange of oxygen and C02, ultimately leading to respiratory failure, asphyxia, and finally death (Shereen, M., et al, 2020).
Many scientists believe that the origins of SARS-CoV-2 trace back to a bat species, known as the Intermediate Horseshoe Bat, Rhinolophus affinis (Boni, M. F., et al, 2020). These pathogen carriers are extremely adaptable to changing environments and have a wide migrational range. Due to urbanization, population growth, and climate change, the displacement of wildlife species from their natural habitats has increased, along with the potential for cross-species viral transfer (Pharrish, R., et al, 2008). Due to the increased exposure of bats to domestically cultivated animal species, virus-host reassortment interactions occurred, yielding mutations that eventually lead to human susceptibility to COVID-19. To understand the potential reach of novel Coronaviruses, predict future viral outbreaks, and develop ways of preventing them, it is vital that global environmental efforts are made to examine cross-species viral transfer in congruence with bat behavior, and the effects of human encroachment on wildlife habitats.
When a pathogen-carrying bat is exposed to another animal, that animal can then become a secondary vertebrate host, and act as a catalyst for a virus and serve to widen its variance rate and species range.
Discussion
These mammals from the order Chiroptera, are extremely adaptable to new environments and despite the growing effects of deforestation on natural bat habitats, these highly evolved pathogen carriers have managed to thrive. Bats can live within colonies of thousands of other bat species and play an important role in the ecological balance of their habitats (Balboni, A. et al., 2012). Bats have an extremely varied diet of insects, fruit, and plants, and are the choice prey of many animals such as birds, snakes, cats, raccoons, weasels, etc. Because of the wide variety in their diet, especially insects, bats are susceptible to exposure to many pathogens. With more than one thousand known species of bats, they also exhibit the largest variety of any vertebrate (Balboni, A., et al., 2012). Meanwhile, bats have changed little since their suspected emergence on the planet, 50-52 million years ago, and are one of the oldest living mammalian species (Balboni, A., et al., 2012). Distinct characteristics have been identified in the research of bat migratory behavior and ability which are informative regarding how easily a particular pathogen or Coronavirus strain can be spread. Bats can travel distances of 200-300 km to almost 2000 km (Balboni, A. et al., 2012). Not only do bats migrate from north to south annually depending on the species, they also migrate within a given season in random directions. It has also been found that bats prefer temperate environments, therefore bats that live in higher elevation areas migrate more extensively (Balboni, A. et al., 2012). However even with seasonal migration, bats also go into deep hibernation when environmental and seasonal challenges become too difficult, for example when food availability is low and/or when lower than normal temperatures affect their habitats. Bats can activate this short and long-term hibernation at will, as a means of saving energy. Consequently, viruses that may be present within the bat, that would otherwise die off, are sustained, and diffuse with other pathogens also present within the bat (Balboni, A., et al., 2012). When bats are forced out of their natural habitats, they often find refuge in construction sites, warehouses, concrete structures, barns, and silos, increasing the chances of exposure to animal species living in close proximity to humans. When a virus-carrying bat is then preyed upon by an animal such as a bird or raccoon, these pathogens then have an opportunity to infect a new host (Balboni., et al, 2012). Bats can also spread whatever viruses they may be carrying through scratching or biting another animal or human, or by releasing airborne particles that are then inhaled by other animals (Balboni., et al, 2012). When a pathogen-carrying bat is exposed to another animal, that animal can then become a secondary vertebrate host, and act as a catalyst for a virus and serve to widen its variance rate and species range (Balboni., et al, 2012). The process that takes place which enables a virus that originated in a bat to infect another species, is referred to as a zoonotic shift. Once humans become infected, it is referred to as a zoonotic disease (Chen. Z., et al 2020). In order for a zoonotic shift to occur, humans must be exposed to either the original host of the virus, referred to as the ‘reservoir host’, or to an animal that contracted the virus from the reservoir host. These secondary vector animals are referred to as ‘intermediate’ hosts. It is possible that a zoonotic shift took place involving Pangolins, an animal whose scales are harvested and used in traditional Chinese medicine (Boni, M. F., et al, 2020). However, in order for a virus to infiltrate the cells of an intermediate host, or a human host, genetic mutations in the virus must occur (Chen. Z., et al 2020). With a higher virulence capability, it has been found that the S (spike) protein of SARS-CoV-2, essential for compatibility with receptor cells of host species, undergoes a rearrangement upon host contact which facilitates its hold onto the Receptor Binding Domain (RBD) of the host cell, as well as its fusion with the host cell membrane (Shereen, M., et al, 2020). Furthermore, the S protein of SARS-CoV-2 is concealed from initial identification by the immune system of the host species, by polysaccharide molecules while cloaking its surface area. Current studies, which focus on the inhibition of these S protein rearrangements, have become essential in the development of antiviral medications (Huang, Y., et al, 2020).
Like all other viruses, RNA viruses like SARS-CoV-2 need a host to replicate.
SARS-CoV-2 has been identified as a member of the coronavirus family based on specific sequence patterns found on its RNA strand, as well as its distinctive crown-like shape which gives coronaviruses their name (Shereen, M., et al, 2020). Like all other viruses, RNA viruses like SARS-CoV-2 need a host to replicate. The RNA strand has all the necessary genetic information to produce the proteins required for the production of new virions; the RNA is copied, eventually leading to the incapacitation of the cells it invades (Shereen, M., et al, 2020). The S (spike) proteins of SARS-CoV-2 protrude outward, enabling the virus to latch on to the ACE2 receptors found in the epithelial skin cells of host species. In order for the ACE2 receptors in the host cell to recognize these protein spikes, they must be extremely specific and compliment the lysine 31 amino acid residue present in the cell (Shereen, M., et al, 2020). The S spikes of SARS-CoV-2 are compatible with Cilia cells, Goblet cells, and Basal cells, all of which are respiratory epithelial cells present in the lungs and trachea (Woolhouse, M., et al, 2012). RNA viruses have the ability to evolve and adapt much faster and more efficiently than other viruses (Woolhouse, M., et al, 2012). However, despite the fact that two-thirds of the viruses that infect humans have been identified as zoonotic in origin, there is a smaller fraction of these zoonotic viruses that can be categorized as ‘specialist’ viruses, such as SARS-CoV-2. Specialist viruses have the potential to infect humans directly and may have evolved alongside humans for hundreds of years (Woolhouse, M., et al, 2012).
Understanding why our exposure has increased and how it has affected the emergence of new viruses is critical. Factors such as deforestation, industrialization, and population growth have intensified the exposure between reservoir host and intermediate host species (Woolhouse, M., et al, 2012). For example, the emergence of the Nipah virus in Malaysia which has been traced to the expansion of fruit farming in close proximity to pig farms is extremely informative regarding reservoir and intermediate host species interaction. Fruit farms in Nipah attracted large numbers of bats, eventually leading to the viral infection of a pig. Because pigs live in close proximity to other pigs and are exposed to humans on a daily basis, the virus eventually spilled over into humans (Pharrish, R., et al, 2008). Luckily, because of many genetic variables, the transmission of many viruses to humans is rare. However, there are certain viruses that have been categorized with the potential to infect a wide range of species, given specific genetic factors as well as, in some cases, the frequency of exposure (Parrish, R., et al, 2008).
Host range is variable among different viruses. For example, the Rabies virus affects a large number of species such as dogs, cats, raccoons, and other mammals, while the Mumps virus has been classified as a single species threat to humans (Woolhouse, M., et al, 2012). It has been found that viruses with a wider range of receptor species are recognized by cell receptors that are highly conserved and present among those various species (Woolhouse, M., et al, 2012). Through the study of receptor proteins, it has been determined that there are few to no examples of viruses that were not specialized, found to infect human cells (Woolhouse, M., et al, 2012). This is the reasoning behind efforts to identify the specific cell receptors that are targeted by a family of viruses, such as coronaviruses. Preventing the receptor cell from recognizing the virus spike, would disable the ability of the virus to attach to the host cell in the first place (Woolhouse, M., et al, 2012).
Population migration and the fragmentation of natural animal habitats have been the blueprint for socio-economic expansion since before the colonization of America (Nichols, B. R., et al, 1999). Sprawl and sub-urbanization are results of new land being settled, followed by the growth of communities, infrastructure development, and the installation of roads. Natural wildlife environments which encase and surround these areas have been subject to the elimination of what were once flourishing natural habitats, as well as the division of landscapes that once provided migrational pathways (Nichols, B. R., et al, 1999). The development of a single village or town is often followed by a city with roadways stretching for miles (Nichols, B. R., et al, 1999). Species with the inherent ability to survive these changes learn to adapt, seeking out new places to find shelter and sometimes new food resources. For the species that don’t survive the decline of their natural habitats or the displacement of their natural food resources, extinction is inevitable (Nichols, B. R., et al, 1999). Large land mammals are the first to suffer the effects of these massive environmental changes. However, the effects of these changes in biodiversity quickly trickle down (Nichols, B. R., et al, 1999). Examples of this are seen in the near extinction of the American Bison, and the total elimination of the Passenger Pigeon (Nichols, B. R., et al, 1999). Fragmentation and the effective development of wildlife patches also result in a loss of diversity among species, preventing some from finding mates, and creating new predators for others (Nichols, B. R., et al, 1999). The breakdown of these natural wildlife habitats also encourages pathogen carriers, such as bats, to expand their migrational territories in search of vital resources and mates. These factors provide ample opportunity for cross-species viral transmission and have undoubtedly played a key role in the emergence of SARS-CoV-2.
Conclusion
Taxonomic relatedness of the host to the virus in combination with access to the host are critical criteria in terms of learning how to prevent the transmission of pathogens from one species to another (Woolhouse, M., et al, 2012). We must build upon the data regarding how viruses have emerged as a result of cross-species viral transmission from bats to farmed animals, and how our encroachment on natural habitats has facilitated these events. The evolution of the SARS-CoV-2 virus follows the patterns described above and based on other similar events of cross-species transmissions, such as SARS-1 and MERS, there is no doubt that the emergence of novel coronaviruses will continue. It is therefore vital that we ascertain new fundamental strategies for the protection of natural habitats and the development of global strategic viral testing and information databases. If we continue to overlook the effects of our expansion into natural wildlife environments and the displacement of natural variations essential to ecological homeostasis, these viruses will continue to emerge, evolve, and bring civilization as we know it to a screeching halt.
References
Parrish, C. R., Holmes, E. C., Morens, D. M., Park, E., Burk, D. S., Calisher, C. H., Laughlin, C. A., Saif, L. J., Daszak, P. (2008) "Cross-Species Virus Transmission and the Emergence of New Epidemic Diseases. Microbiology and Molecular Biology Reviews". Retrieved from https://mmbr.asm.org/content/72/3/457.short
Balboni, A., Battilani, M., Prosperi, S. (2012). "The SARS-like coronaviruses: the role of bats and evolutionary relationships with SARS coronavirus." New Microbiologica, 35, 1-16. Retrieved from http://www.newmicrobiologica.org/PUB/allegati_pdf/2012/1/1.pdf
Chen, Z., Senft, R. (MARCH 22, 2020) "COVID-19 and Emerging Viral Diseases: the journey from animals to human." BLOG. Harvard University the Graduate School of Arts and Sciences. Retrieved from http://sitn.hms.harvard.edu/flash/2020/covid-19-emerging-viral-diseases-journey-animals-humans
Woolhouse, M., Scott, F., Chase-Toppin, M. (October 19, 2012). "Human viruses: discovery and emergence." Philos Trans R Soc Lond B Biol Sci. 367(1604): 2864–2871. Retrieved from https://www.ncbi.nlm.nih.gov/pmc/articles/PMC3427559
Sheeran, M. A., Khan, S., Kazmi, A., Bashir, N., Siddique, R. (2020) "COVID-19 infection: Origin, transmission, and characteristics of human coronavirus." Journal of Advanced Research. Vol. 24, 91-98. Retrieved from https://www.sciencedirect.com/science/article/pii/S209012322030054
Nichols, B. R. (April 1999) "The Destruction of Wildlife Habitat by Suburban Sprawl and the mitigating effects of Land Use Planning". Proceedings of the 1999 Northeastern Recreational Research Symposium. 177-180.
Huang, Y., Yang, C., Xu, Xf. et al. (2020) "Functional Properties of SARS-CoV-2 spike protein: potential antivirus drug development for COVID-19." Acta Pharmacol Sin. Vol. 41, 1141-1149. Retrieved from https://www.nature.com/articles/s41401-020-0485-4#citeas
Boni, M. F., Lemey, .P., Jiang, X., Tsan-Yuk Lam, T., Perry, B. W., Castoe, T. A., Rambaut, A., Robertson, D. L. (2020) "Evolutionary Origins of the SARS-CoV-2 sarbecovirus lineage responsible for the COVID-19 pandemic." Nature Microbiology. Vol. 5, 1408-1417. Retrieved from https://www.nature.com/articles/s41564-020-0771-4
Commentaires